Eating disorders represent a growing concern in Mexico, with a notable increase in recent years. Anorexia, bulimia, and obesity pose significant challenges to public health, especially among youth. To better understand the biological bases of these disorders, a study focused on the brain circuits of motivation and reward has been undertaken, exploring the role of EBF-2 expressing neurons.
The primary goal of this research was to characterize the motivation and reward circuits in 129SV-Ebf2-tau.GFP mice. As such, it was crucial to analyze the patterns of physiological and hedonic intake in Ebf2 knock-out mice, contrasting them with heterozygous and wild-type mice.
Theoretical Framework
- Physiological and Hedonic Intake: a distinction is made between physiological intake, motivated by caloric need, and hedonic intake, related to the pleasure of eating. These processes are regulated by motivation and reward circuits in the brain.
- Motivation and Reward Circuits: The limbic system, which includes structures such as the hypothalamus and amygdala, plays a crucial role in regulating motivated behavior and reward-seeking. The lateral hypothalamic nucleus and the parabrachial complex are specific regions of interest in this study.
- Early B-Cell Factor 2 (Ebf2): This transcription factor plays an important role in neuronal development and is expressed in areas of the brain associated with motivation and reward. The gene for this factor was genetically modified using homologous recombination by replacing the EBF-2 protein instructions with fluorescent tau-GFP protein instructions. Additionally, this gene is also present in humans.
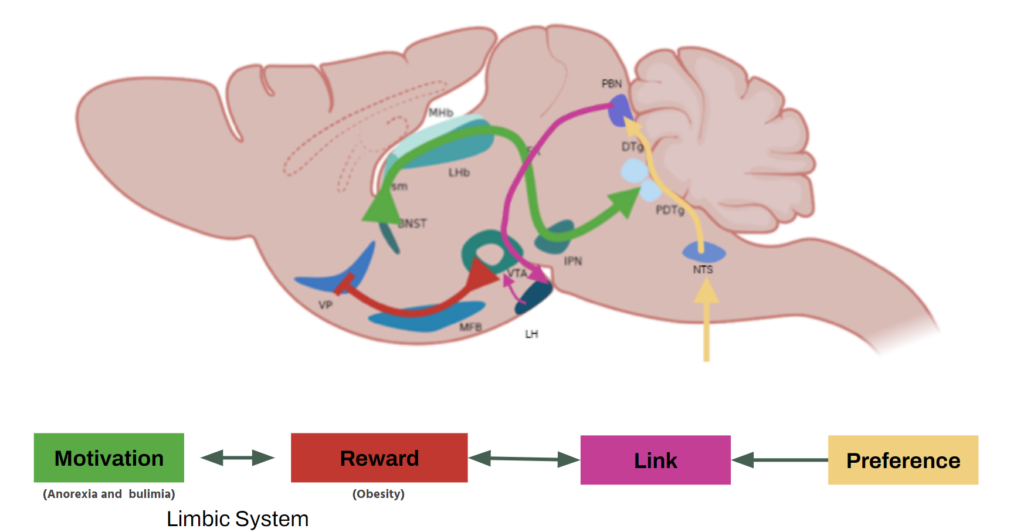
Methodology
Subject mice had to be chosen very carefully due to colony size. they needed to be from the same litter and a specific postpartum period. Prior observations, pointed out some phenotype differences between the three groups. Knock-out (KO) mice often exhibited dwarfism and a darker coat, facilitating their identification. Nonetheless, genotypification was required to ensure proper selection for the testing sample.
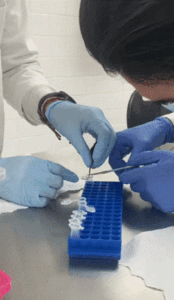
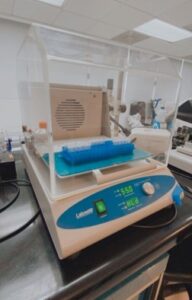
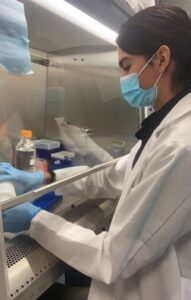
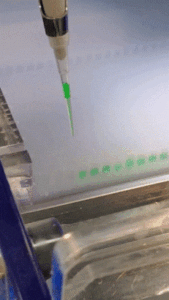
DNA extraction was a crucial process for this research, involving the extraction of genetic material from samples obtained from the subjects’ tails that went through a tissue lysis protocol to extract the genetic information.
PCR was used to amplify the genetic material, employing primers for EBF-2 and tau-GFP to identify the subject’s genotype. Gel electrophoresis was utilized to analyze and characterize nucleic acids, separating nucleotide chains by size. EBF-2 is a longer chain and therefore is the left column. tau-GFP is a shorter molecule and can travel further through the gel. Testing could not be performed under the same due to proximity in the molecular weight as they can overlap.
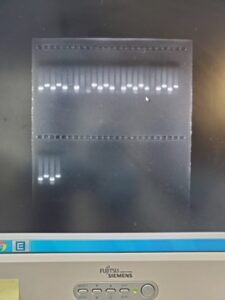
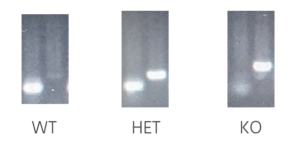
The experimentation process was as follows:
- The mice were placed in individual cages, and food was removed overnight.
- The next day, all mice were weighed while fasting, and the data was recorded in an Excel spreadsheet.
- Each mouse’s food was placed in a Petri dish. The weight of the food was recorded in Excel.
- The mouse was allowed access to the food for 30 minutes. At the end of the 30-minute period, both the mouse and the food were weighed. Changes in weight and feeding patterns according to the mouse genotype were recorded in Excel.
- The mouse was allowed to continue eating for an additional 60 minutes. At the end of the 60-minute period, the mouse and food were weighed again, and the data was recorded in the logbook for transcription into Excel.
This sequence of experimentation was conducted with both the normal mouse food to analyze physiological intake and with the “more pleasurable” food, which was higher in sugars compared to regular food, to evaluate hedonic intake. At least one full day was allowed between physiological intake experimentation and hedonic intake experimentation for mouse rest.
To optimize the video capture process, it was decided to use a 360-degree camera, the Insta 360 One R model, which allowed recording a complete sphere rather than a single plane.
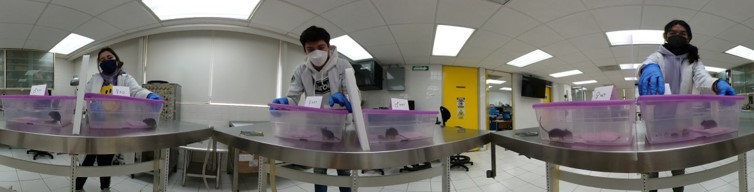
After completing each set of experiments, subjects were euthanized for brain and spine extraction via meticulous microsurgery to facilitate further research. Sagittal sections of the brain were then captured using the Zeiss Axioscope 5.
Results
After doing some statistical analysis data showed significant differences in intake patterns between mice with alterations in the EBF-2 gene and wild-type mice. Increased continuous intake is observed in knock-out mice, suggesting dysfunction in the reward circuit. Additionally, differences in latency and duration of intake are found, indicating an altered response to food stimuli in mice with genetic alterations.
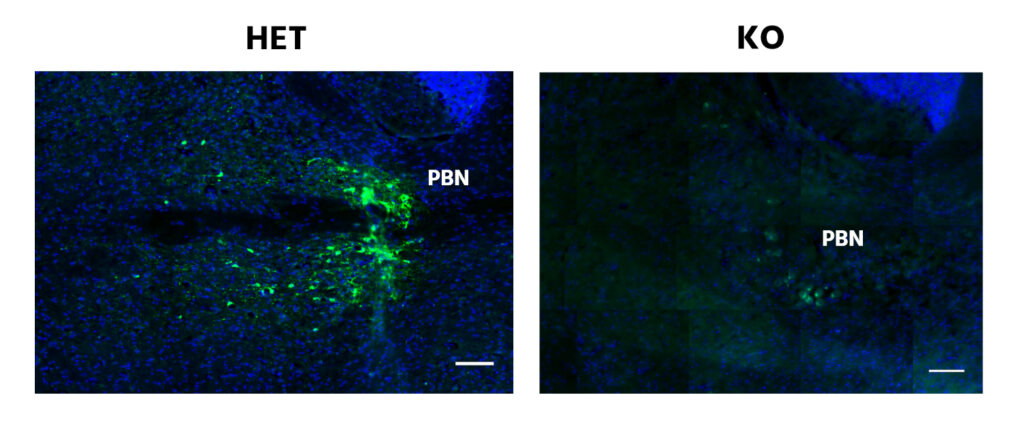
Fluoromicroscopy images of the Parabrachial Nucleus show that KO subjects possess a diminution of the reporting protein tau-GPF by the EBF-2 promoter compared to its HET counterparts. This pattern is also present in the Lateral Hypothalamic Nucleus but to a lesser degree.
Conclusions
This study provided valuable information on the neurobiological mechanisms involved in eating disorders. Characterizing motivation and reward circuits offers new perspectives for the development of therapies targeting these disorders. However, further research is needed to fully understand the complexity of these phenomena and develop effective interventions.
Leave a Reply